Volume 1 - Year 2015 - Pages 7-12
DOI: TBA
Operational and Technical Parameters Influencing the Design of Hybrid Drive Public Transport Vehicles
Dalibor Barta1, Martin Mruzek2
1University of
Zilina, Department of Mechanical Engineering
Univerzitna 1, 010 26 Zilina, Slovakia
dalibor.barta@fstroj.uniza.sk
2University of Zilina, University Science Park
Univerzitna 1, 010 26 Zilina, Slovakia
mruzek@uniza.sk
Abstract - Ecology of transport is one of the most frequent topics of professional community nowadays. Sustainable transport requires new solutions in vehicle design, especially in their propulsion. For the design of public transport vehicles drives it is important to work with parameters close to the real conditions. This helps to find the best conception of the drive and so reduce the production of air pollutants and the consumption of energy. Energy intensity of the vehicle is usually determined on the basis of a driving simulation based on driving cycles for measuring of harmful emissions and fuel consumption. These simplified driving cycles do not include the grading resistance therefore they are insufficient to determine certain specific conditions. Compilation of driving simulation based on real driving cycles measured during the driving of the vehicle in urban traffic seems to be more appropriate for specific vehicles, such as buses for public transport are. This paper shows to what extent the use of standard driving cycles for the design of hybrid drives, respectively electric drives of vehicles is consistent with real conditions.
Keywords: Real driving cycles, Public transport, Energy intensity, Hybrid drive, Measurement, Bus lines.
© Copyright 2015 Authors - This is an Open Access article published under the Creative Commons Attribution License terms. Unrestricted use, distribution, and reproduction in any medium are permitted, provided the original work is properly cited.
Date Received: 2015-07-07
Date Accepted: 2015-12-06
Date Published: 2015-12-22
1. Introduction
According the Habarda [1] theigh efficiency of vehicles drives, low level of harmful emissions and low level of noise are basic requirements imposed on public transport vehicles. However the conventional drives with internal combustion engines are no longer able to successfully meet these requirements. The importance of the use of electric and hybrid vehicles in city public transport therefore increase.
Chan et al. [2] considered transit buses to be the best candidates for hybrid application because they normally operate on predictable routes with frequent starts and stops. A hybrid propulsion system is better suited for transit buses than private cars because they operate at lower speed, limited acceleration, less road grades and ample space available for batteries.
The information about intended use of some vehicles and real condition at place of their operation are necessary to properly design the drive of this vehicle. Efficiency of utilization of electric and hybrid drive systems depends on their right dimensioning and on the appropriate combination of their subsystems [3].
In order to reduce the vehicle emissions and energy consumption, some systems for the accumulation of kinetic energy from deceleration and braking which would be otherwise wasted by heat as well as systems which switch off the combustion engine while vehicle standing, as stop/start system, are currently designed. Proposals of similar devices as well as the hybrid drives are usually based on driving cycles for determining of harmful emissions and fuel consumption, such as Braunschweig bus cycle, Millbrook Westminster London Bus cycle - inner London or BP bus cycle [4]. These cycles are however simplified. They don't contain the grade resistance or real progress of braking deceleration. In the normal traffic, there is often slow down, respectively a short-term braking, which can be only a minor source of recovered energy, as mentioned Huilong et al. [5]. For the purpose of comparison of standardized and real driving cycles several measurements of real driving cycles in different cities of Slovakia, Poland and Czech Republic.
As Gao et al. [6] introduced the dynamic interactions among various components and the multidisciplinary nature make it difficult to analyze a newly designed hybrid electric vehicle. Each of the design parameters must be carefully chosen for better fuel economy, enhanced safety, exceptional drivability, and a competitive dynamic performance-all at a price acceptable to the consumer market. Prototyping and testing each design combination is cumbersome, expensive, and time consuming. Modeling and simulation are indispensable for concept evaluation, prototyping, and analysis of Hybrid electric vehicle. Therefore the simulation of hybrid drive of the bus based on the values from real driving cycles was performed.
2. Measurement of real driving cycles
Measurements of speed, distance, acceleration, breaking point and elevation were performed. The measuring equipment consisting of measuring unit DAS 3 (Data Acquisition System) with contactless speed sensor, GPS (Global Positioning System) receiver NaviLock NL-302U and CORRSYS-DATRON Pedal Force Sensor (Fig. 1.) were used for collecting of the data on vehicle movement.
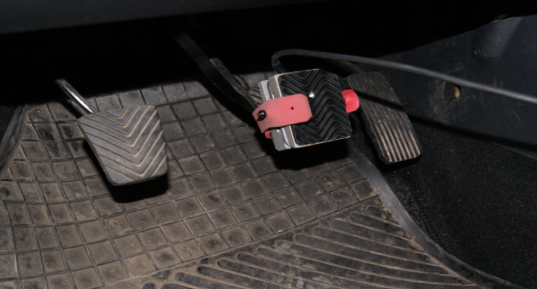
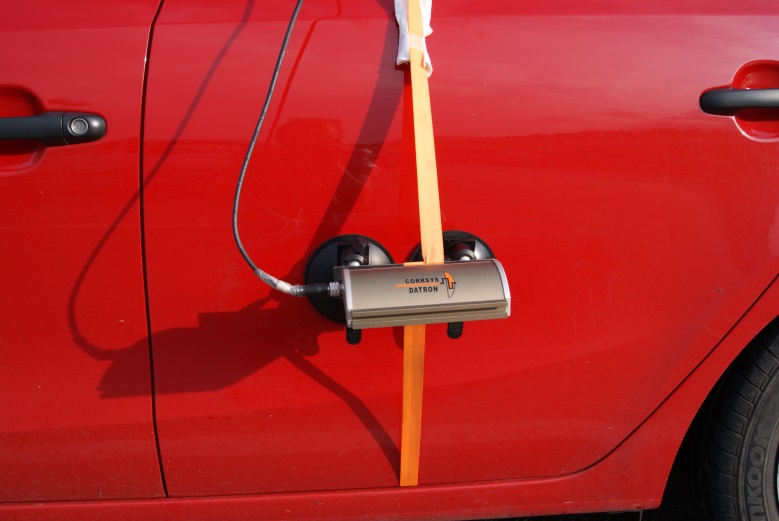
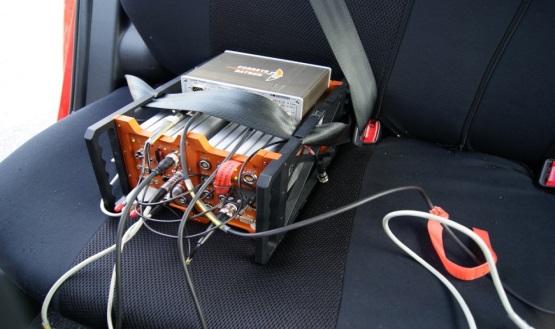
The right timing of the measurement of real driving cycles is very important. As stated in [7] there are big differences in the traffic during the day and during the week too.
The chart on the Figure 2 shows how the number of trips in progress changes over the course of 24 hours for different days of the week. The chart is presented as an index, which compares the number of trips in progress per hour on a weekday, a Saturday and a Sunday with the average number of trips in progress per hour across all hours in a week.
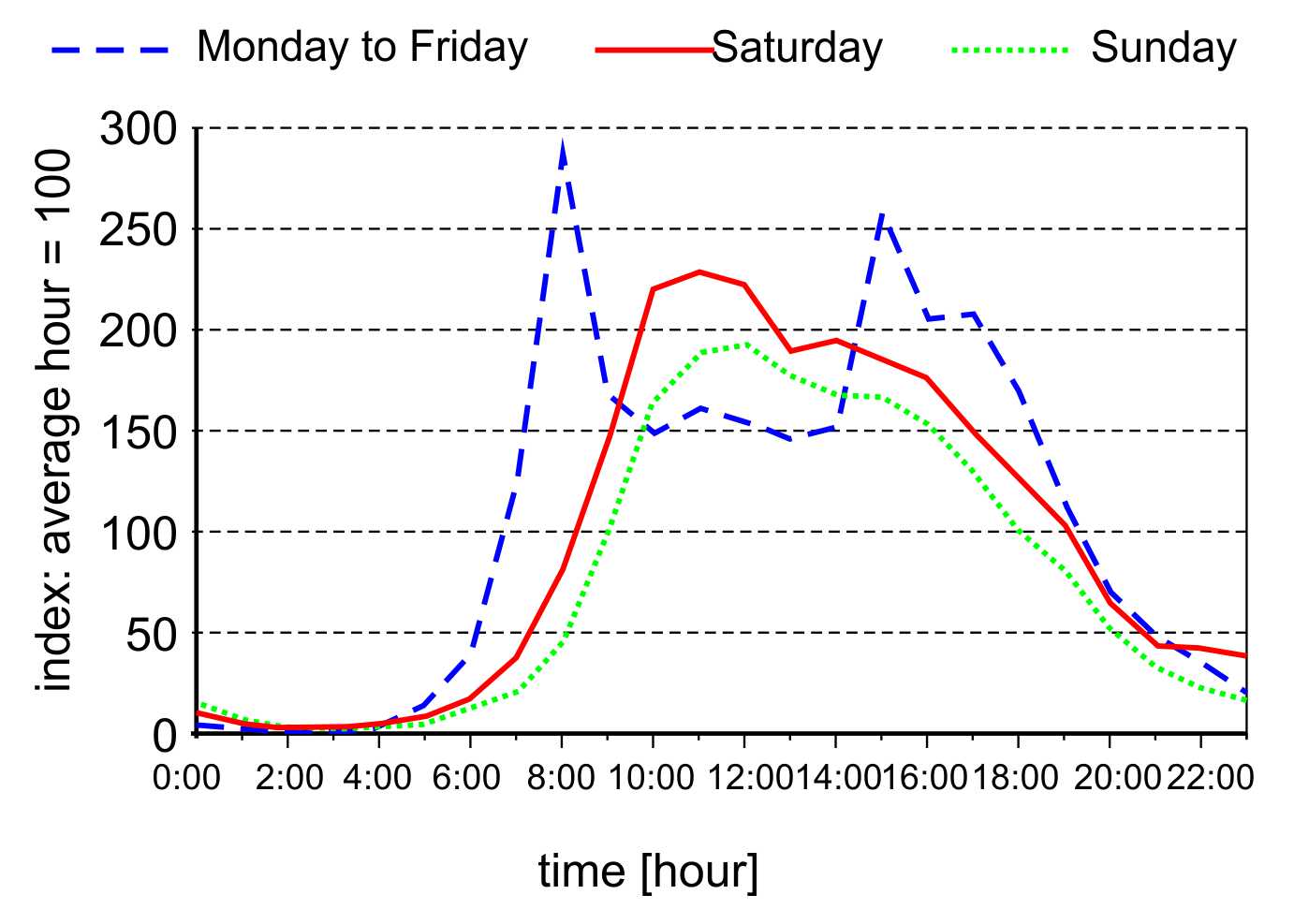
The chart shows two peak hours for the number of trips in progress on a weekday (Monday to Friday). The first (and highest peak) is in the morning between 8:00 and 8:59 when there are nearly three times as many trips in progress when compared with the average hour. The second peak is in the afternoon between 15:00 and 15:59. Both peaks are driven by education related trips. There is only one peak hour for trips in progress at the weekend. On Saturday the peak hour is between 11:00 and 11:59 and on Sunday the peak hour is between 12:00 and 12:59. Overall the number of trips in progress on a Sunday is 24% lower when compared with the average day. The busiest day of the week in terms of the number of trips was Friday with 150 trips per person per year.
Based on these facts and because it is assumed that electric and hybrid vehicles are used mostly for transportation to job and shopping by their owners in middle European region, the measurements were performed in real time of their use, in the time of afternoon rush hours from 14:00 to 16:30 hrs. This time, typical for our region, is characterized by growing number of traffic congestions and longer standing at intersections. The most energy-demanding regimes of frequent acceleration and braking were recorded by this way.
One of the most varying factors affecting the value of required vehicle performance is the elevation profile of the city. Selected elevation profiles recorded during the measurement in Slovakia, Poland and Czech Republic show the Figures 3 to 5.
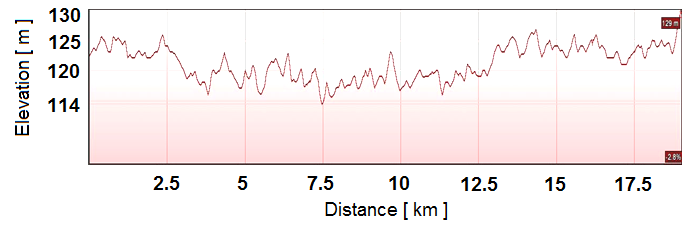
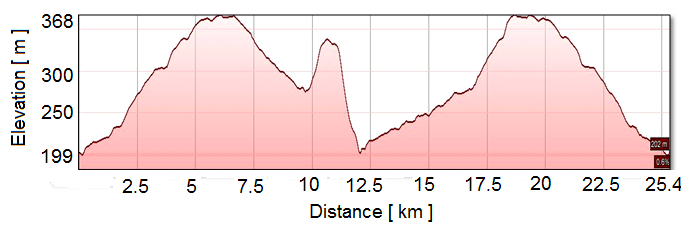
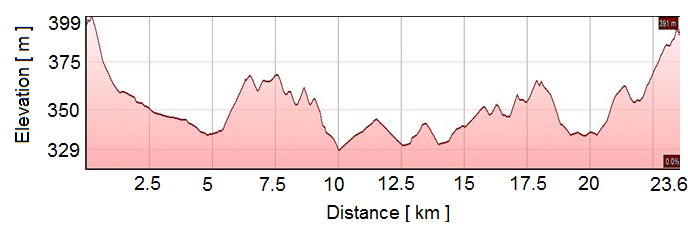
In Zilina, there were measured driving cycles of three bus lines (No. 21, 27 and 31) of the Zilina public transport. Table 1 shows the basic characteristic parameters of these driving cycles.
Figure 6 represents the elevation profiles of the line routes 27 and 31. Results of driving cycles analysis shown relatively big height differences in individual elevation profiles. Significant differences in these values were recorded also between individual lines within the city. The real driving cycles of specific bus lines were measured in both directions, back and forth, to ensure the objectivity of measurements.
Table 1. Characteristic parameters of real driving cycles of city transport bus lines in Zilina.
Bus Line | Distance | Average Speed | Time of cycle |
[ km ] | [ km.h-1 ] | [ min ] | |
Line 21 | 8.9 | 22.19 | 24 |
Line 27 | 15.5 | 29.96 | 31 |
Line 31 | 13.7 | 26.43 | 31 |
Table 2. Descent and ascent characteristic parameters of real driving cycles of city transport bus lines in Zilina.
Bus Line | Max. descent | Max. ascent | Average ascent | Average descent |
[ % ] | [ % ] | [ % ] | [ % ] | |
Line 21 | -16.9 | 12.6 | 1.3 | -1.3 |
Line 27 | -10.6 | 15.4 | 3.3 | -2.3 |
Line 31 | -15.2 | 10.0 | 1.7 | -2.3 |
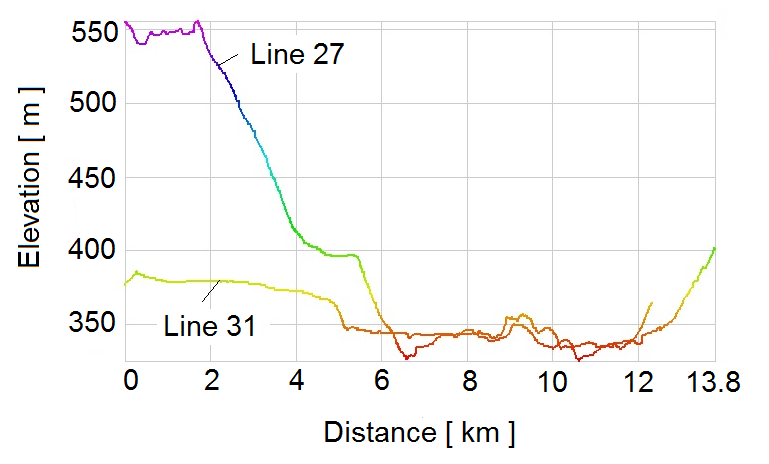
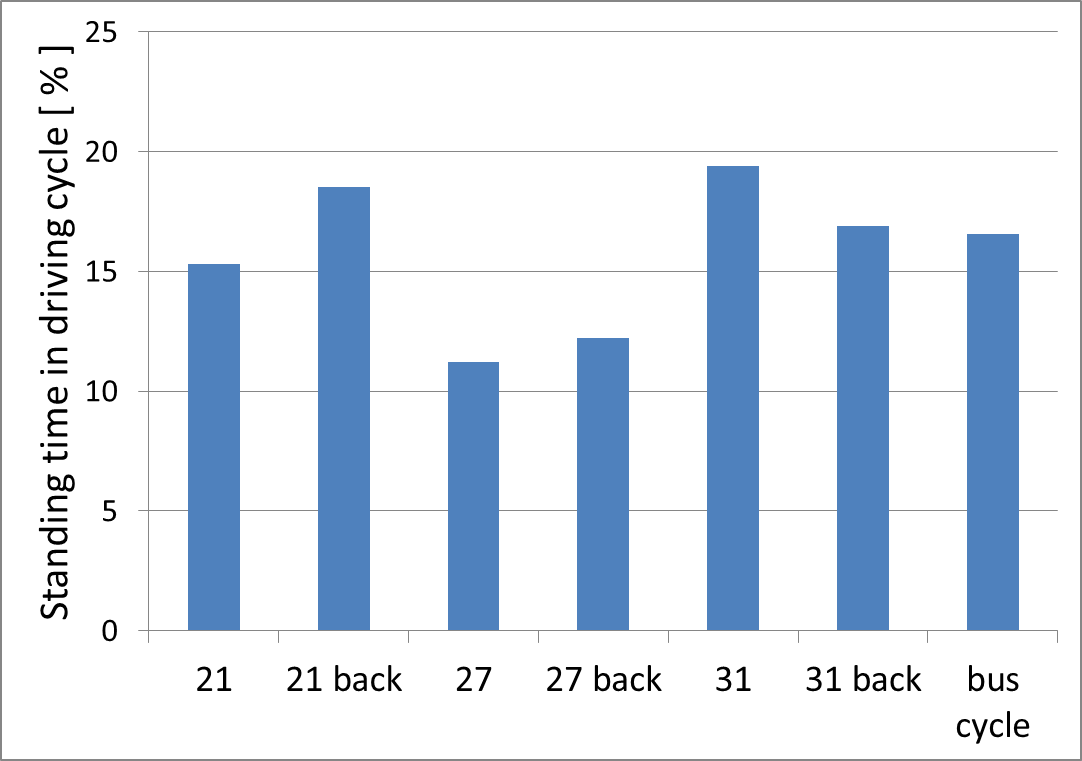
Analysis of the standing time (defined as a time when the speed and the acceleration of the vehicle is equal to zero - bus stops, intersections, in traffic jam etc.) useful for the stop/start system design comparing the standing time of measured lines with Braunschweig bus cycle is shown in the Figure 7. As can be seen, the measured values oscillate around the value of Braunschweig bus cycle.
3. Simulation of the hybrid bus drive
According to a study by Rahman et al. [8] among many different hybrid configurations, the two generally accepted classifications are series and parallel. The parallel hybrid is more efficient satisfying high road power demands (high speed or acceleration), while series is more efficient for large energy demands. For public transport purposes, where large weight is of more concern than high acceleration, the series propulsion strategy becomes more efficient and simpler. On the basis of that the serial hybrid drive of a bus was chosen as a subject of the simulation. The simulation was done in Matlab Simulink for 3 categories of buses (minibus, 10m long bus and 12m long bus) with specific parameters for different occupancy 25, 50, 100% [9]. Measured actual driving cycles were used as an input to the simulation. Required power of the bus was calculated based on selected parameters and the real driving cycle.
Four variants of serial hybrid drive with different parameters of key components were simulated. Two types of Li-ion batteries with different capacity, two types of internal combustion engines with different power, electric traction motor and two types of generators were used [10]. Behaviour of individual components of the hybrid system was simulated based on the control strategy. Internal combustion engine was operated in one revolution mode with the best specific fuel consumption [11], [12]. The operation of the combustion engine was controlled by state of charge (SOC). If the SOC reached preset lower limit the combustion engine was turned on. When SOC reached preset top limit where the battery was fully charged, the combustion engine was turned off [13].
The results of simulations based on the values from real measured driving cycles can be seen on next figures. Analysed were the recoverable energy from the driving cycle Erek, energy E and average power Paver required to pass the cycle.
As Figure 8 shows, energy required to pass the cycle E and the value of recoverable energy Erek increase with the occupancy of the vehicle. There are some differences between values obtained at measurement in the direction back and forth caused by changes in the number of descents and climbs on the route (see elevation profile on Figure 6). This fact leads to the need to take into account the measured values from both directions of the bus line route, when design the bus drive.
The Figure 9 shows, that the maximum difference in the values of average power at comparison with all three lines in both directions represent for example at the 12m long bus with the weight of 12855kg up to 25kW, comparing the lines 21 and 27. This value represents 78% of the average power needed for the passing the line 21.
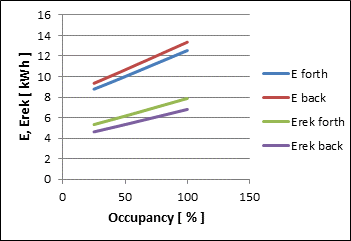
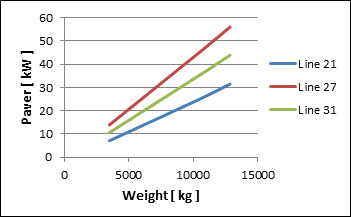
The course of the energy which could be re-used from the driving is represented by the Figure 10. As show these pictures, the amount of recoverable energy depends on the various parameters of the bus line route such as profile, distance and amount of decelerations. Due to the similar elevation profile, number of stops and common part of the route the amount of recoverable energy can be very similar for some city lines (line 27 and 31). But it can be also very different for line routes led in different conditions (line 21).
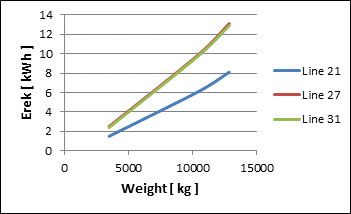
The same situation is with the energetic requirements on the buses. The energetic requirements on the bus drives can be very diverse in one city. These results point to the need of individual approach to the proposal of hybrid buses drives for each bus line.
The fact that the route and timetable of drive throughout the day is known is big advantage at the design of public transport buses. With this knowledge it is able to configure the hybrid drive to operate as efficiently as possible. One of the assumptions is that the bus will start from the depot with fully charged battery and when return to the depot the SOC is low as possible so that the bus can be charged in depot.
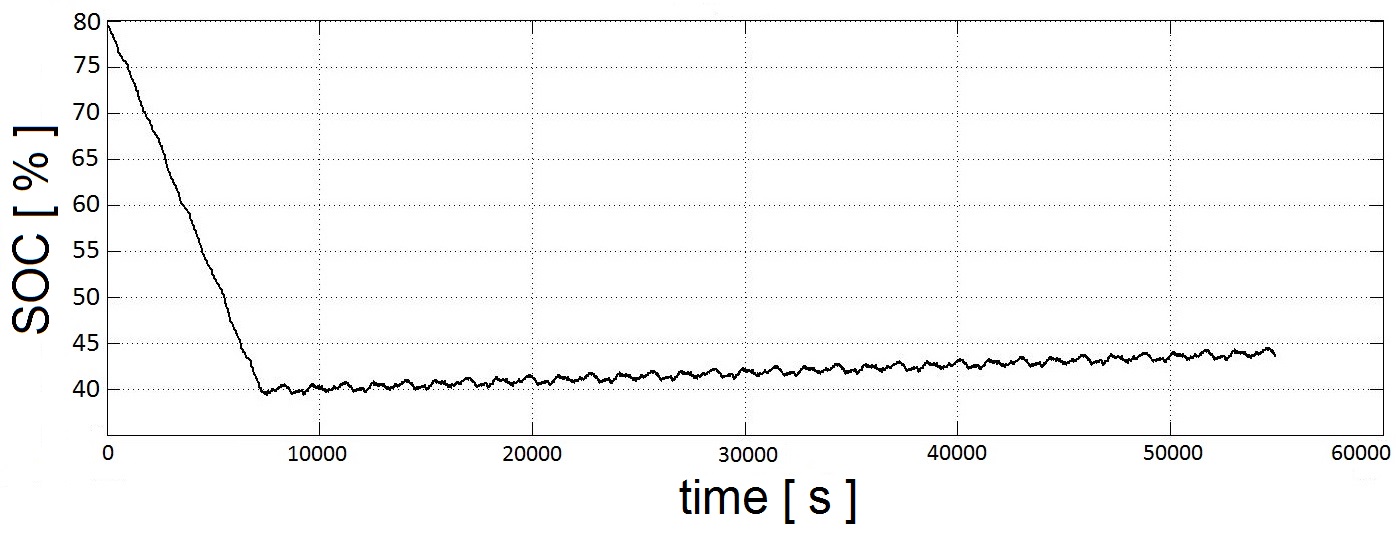
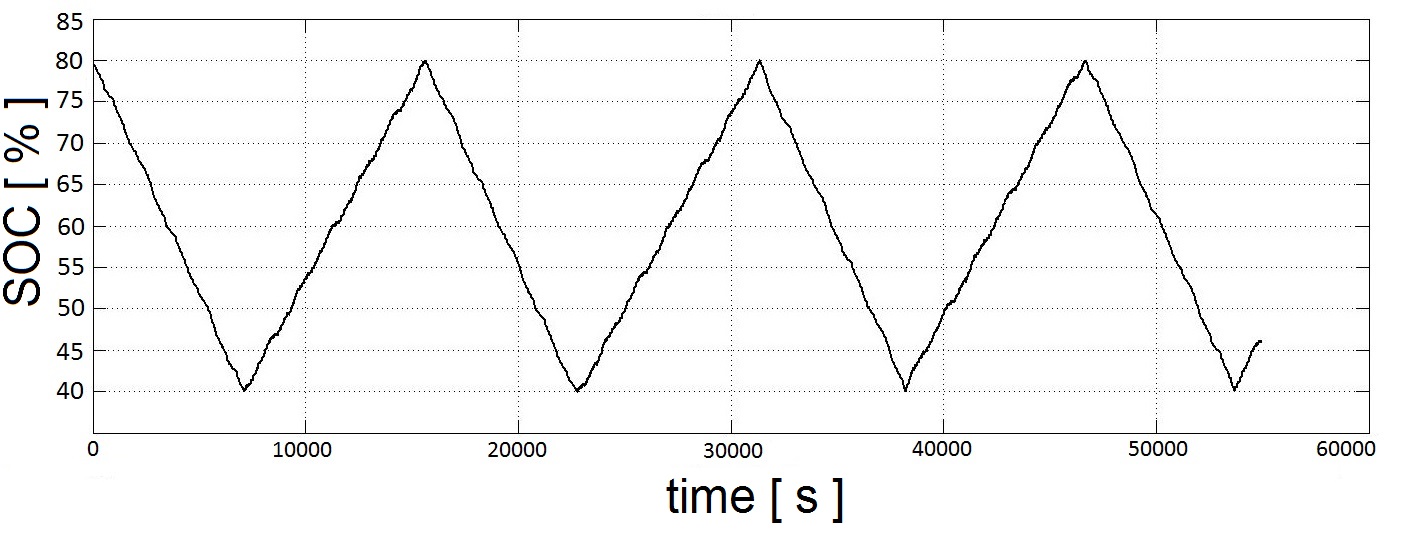
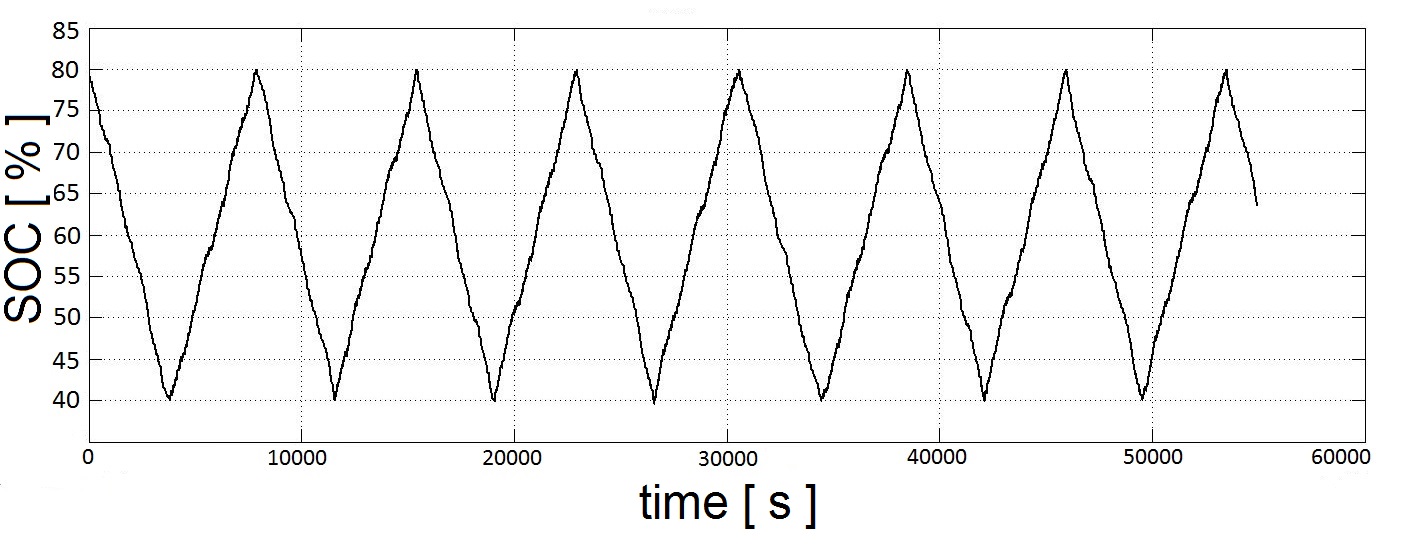
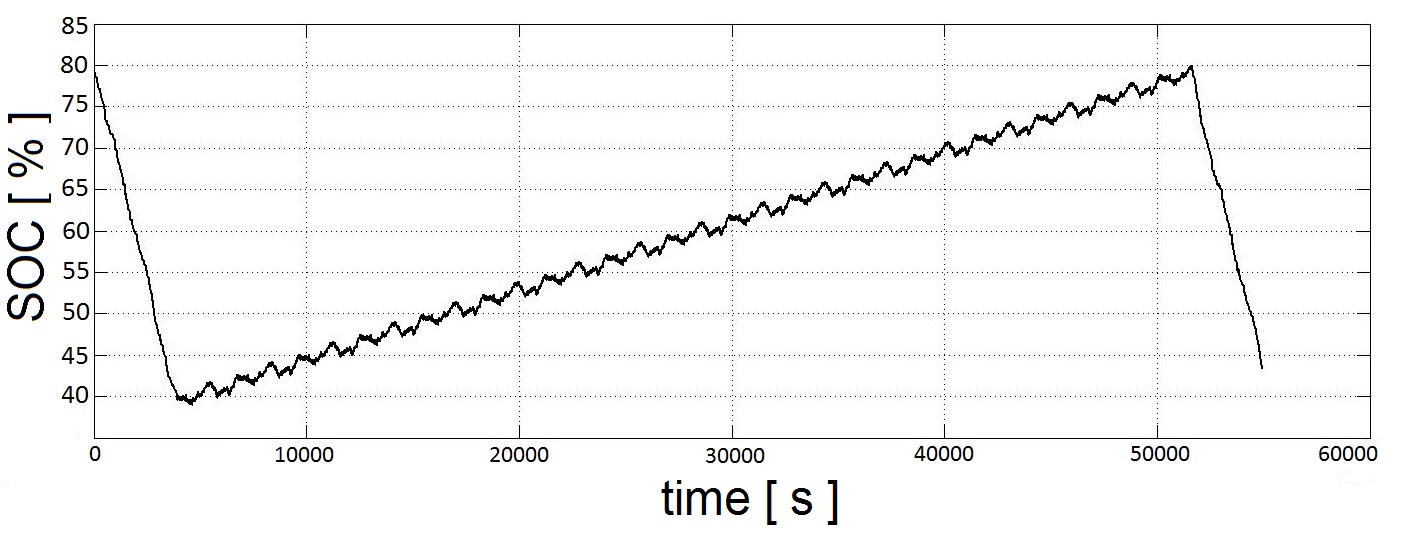
In this case the battery will be charged from the public electricity network and so it is possible to reduce production of emissions that would be otherwise created by combustion engine during battery charging. Second key factor is dimensioning of combustion engine size. As shows the simulation the battery charging time depends on the size of the internal combustion engine and battery capacity (Figures 11-14). Combustion engine in the simulation run in optimum conditions with the lowest specific fuel consumption. Engine power should be never less than the average power from the real driving cycle because in this case cannot be guaranteed that the hybrid drive has sufficient power for drive. Especially in city centres is big emphasis on the production of harmful emissions. Therefore, it is important to propose the hybrid drive and charging management so that the batteries will be charged enough before entering into selected area of the city and will have sufficient capacity for driving only on electric drive.
4. Conclusion
Correct design of a hybrid or electric drive depends on the appropriate choice and arrangement of individual elements in terms of their size, performance and capacity. Driving cycles measured in real traffic capture specific driving conditions of the city, respectively specific line characterized by the number of starts and stops, grade or driving speed and better characterize the real situation of the traffic in the city. Impact of the organization and the management of the traffic in the city greatly affect the ratio of the time of standing and driving.
The concept of design of a modular hybrid drive with adaptive management has its justification in the design of urban buses, because it allows complete individualization of buses with hybrid drive with an emphasis on ensuring minimum impact on the environment and reducing of economic costs.
Acknowledgements
This paper is supported by following projects:
University Science Park of the University of Zilina (ITMS: 26220220184) supported by the Research&Development Operational Program funded by the European Regional Development Fund and projects ITMS 26110230107, ITMS 26110230117, VEGA 1/0927/15 Research of the use of alternative fuels and hybrid drives on traction vehicles with aim to reduce fuel consumption and air pollutants production.
References
[1] D. Habarda, "Mestska hromadna doprava (Transl.: Public Transport)," Bratislava, Alfa, 1984, pp. 489-490. View Article
[2] C. Chan and K. Chau, Modern Electric Vehicle Technology, New York: Oxford University Press, 2001. View Article
[3] M. Ehsani, et al, Modern Electric, Hybrid Electric and Fuel Cell Vehicles, 2nd ed., Florida: CRC Press, 2010. View Article
[4] T. Barlow, et al, A reference book of driving cycles for use in the measurement of road vehicles emission, Berkshire: TRL Limited, 2009. View Article
[5] Y. Huilong, X. Junqiang and C. Yongdan, "Research on Shift Schedule of Hybrid Bus based on Dynamic Programming Algorithm," in 15th International IEEE Conference on Intelligent Transportation Systems Anchorage, Alaska, 2012. View Article
[6] D. Gao, et al, "Modeling and Simulation of Electric and Hybrid Vehicles," Proceedings of IEEE, vol. 95, no. 4, pp. 729-745, 2007. View Article
[7] Dept. of Transport, "National Travel Survey: 2012," 30 July 2013. [Online]. Available: https://www.gov.uk/government/uploads/system/uploads/attachment_data/file/243957/nts2012-01.pdf. [Accessed 12 Jan. 2014]. View Article
[8] Z. Rahman, K. Butler and M. Ehsani, "Design studies of a series hybrid heavy-duty transit bus using V-ELPH 2.01," in IEEE 49th Vehicular Technology Conference, Houston, 1999. View Article
[9] H. Kristína, "Posterus," 02 February 2011. [Online]. Available: http://www.posterus.sk/?p=9876&output=pdf. [Accessed 20 September 2014]. View Article
[10] D. Kalincak and L. Bartik, "The possibilities of fuel economy - the hybrid traction propulsion," Prace Naukowe. Transport: srodki i infrastruktura transport, vol. 98, pp. 225-235, 2013. View Article
[11] P. Punov and T. Evtimov, "Possibilities of using a standalone ECU for gasoline engine control in test bed running conditions," in 14th International scientific-technical conference trans&MOTAUTO'08, Bulgaria, 2008.
[12] A. Rimkus, et al, "Research of hydrogen influence for gas bus ecological and economic parameters," in Transport Means 2012 - 16th international conference, October 25-26, 2012, Kaunas University of Technology, Lithuania, 2012.
[13] S. Dewenterl, A. Binder and M. Strauch, "Simulation model for a serial hybrid bus and impact of energy management on fuel consumption," in IEEE Vehicle Power and Propulsion Conference, Seoul, 2012. View Article